It’s become fairly common knowledge that Yellowstone National Park, in addition to being incredibly beautiful, is sitting on top of an enormous supervolcano that catastrophically erupts every few hundred thousand years. Unlike normal volcanoes, which tend to produce large cone-shaped mountains made of ash and lava, supervolcano eruptions (defined being at least an 8 on the Volcanic Explosivity Index, meaning at least 1000 cubic kilometers of rock are released) are so enormous that the ground beneath the eruption collapses, forming a huge depression known as a caldera. Yellowstone Park largely exists within the Yellowstone Caldera, formed during Yellowstone’s last large eruption 640,000 years ago.
(Not every Yellowstone eruption is a large, catastrophic one. Its most recent eruption, 70,000 years ago, was much smaller).
The supervolcano is the result of the Yellowstone hotspot, an area of high temperature within the earth’s crust suspected to be caused by large mantle plume, an upwelling of the earth’s hot, molten mantle. The Yellowstone hotspot has gradually moved east over time as the earth’s tectonic plates have shifted, leaving behind a series of calderas that mark each large-scale eruption.
Earth hasn’t seen a supervolcano eruption in recorded history, but if one were to occur the effects would be catastrophic. From “The Precipice”
Everything within 100 kilometers of the blast is buried in falling rock, incandescent with heat. Thick ash rains down over the entire continent. When the Indonesian volcano, Toba, erupted 74,000 years ago, it covered India in a blanket of ash a meter thick and traces were found as far away as Africa…The dark volcanic dust and reflective sulfate aerosols unleashed by the Toba eruption caused a “volcanic winter,” which is thought to have lowered global temperatures by several degrees for several years. Even the much smaller eruption of Indonesia’s Mount Tambora in 1815 (less than a hundredth the size) caused a global cooling of 1°C, with places as far away as the United States suffering crop failure and June snows in what became known as the “year without a summer
The volume of ash ejected into the atmosphere would be similar in magnitude to the asteroid impact that killed the dinosaurs (along with 75% of all plant and animal species), and have similar effects:
The effects may be roughly comparable to those of the one-to ten-kilometer asteroids, with major global crop failures lasting for years on end. Since the world only has about six months of food reserves, there is a possibility that billions of people could starve and that civilization could suffer a global collapse
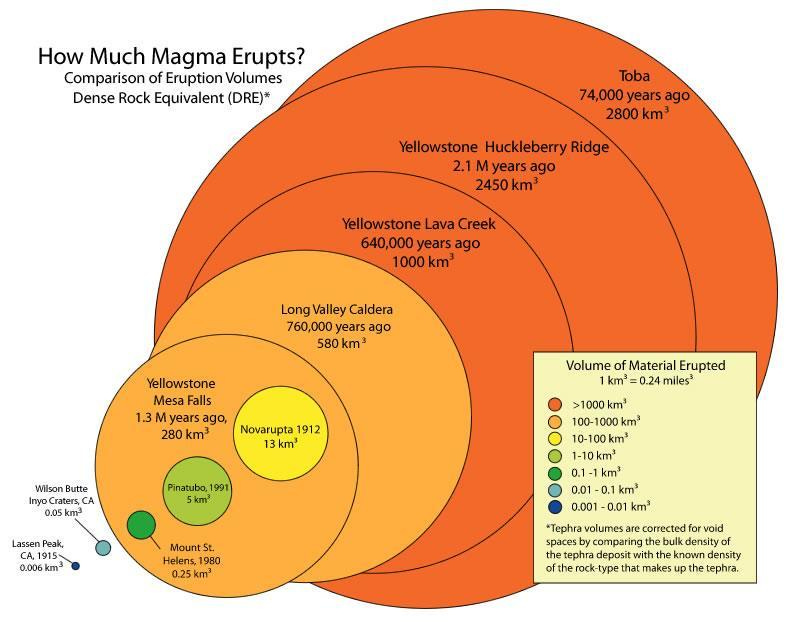
Unlike most catastrophic risks, however, addressing the risk of a supervolcano eruption also presents an opportunity. A volcano is fed by a magma chamber, a large mass of liquid and semi-liquid rock deep below the surface. In Yellowstone’s case, there are two magma chambers: a smaller, more liquid one 4 to 14 kilometers below the surface which is about 10% molten rock, and a larger one 20 to 45 kilometers below the surface which is about 2% molten rock (though more recent analyses suggest a higher melt fraction). Combined, these magma chambers are about 56,000 cubic kilometers in volume, and the lower chamber is thought to grow at a rate of about 0.3 cubic kilometers per year.
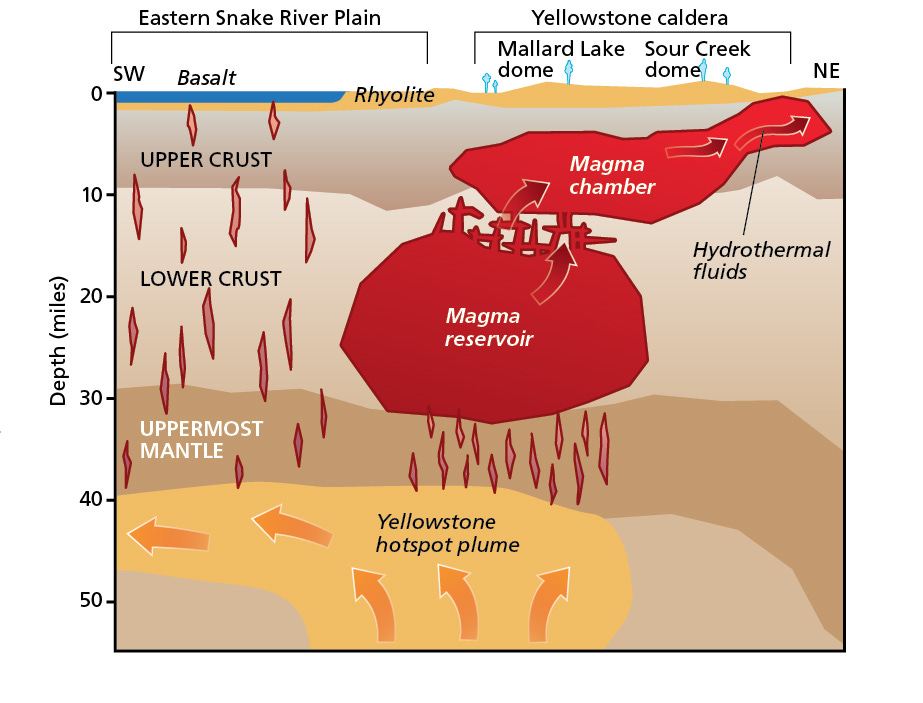
If heat could be bled off of the magma chambers, cooling and solidifying them, not only would that (theoretically) stop the volcano from erupting, but the heat could be used to generate electric power with a geothermal energy plant. Depending on the size of the plant and how quickly it bled off the heat, this could be a very large amount of electricity - enough to power the entire US.
A brief note on geothermal energy
The earth contains an enormous amount of heat - the crust alone has 41 times more thermal energy than all known petroleum and nuclear fuel reserves combined. Geothermal energy plants tap this heat to generate electric power. Though there are different technologies available, they all use the same basic principle - a plant draws up heated water (or some other heat-conducting fluid) from within the earth, and uses it to create steam to drive a generator. The fluid then gets pumped back into the earth to absorb more heat.
Historically, geothermal energy plants have been limited to places where heat from the interior was unusually close to the surface, and there was a ready supply of heated water flowing through permeable rock that could be tapped for power. The largest geothermal plant in the world, The Geysers in California, is built on the site of large naturally occurring hot springs. But geothermal energy is available anywhere if you can drill deep enough and find a way to pump fluid in and out. Increasingly, people are investigating enhanced geothermal systems (EGS), which can create geothermal power nearly anywhere by drilling very deeply and creating permeable rock with fracking techniques.
As you might expect, the magma chamber beneath Yellowstone releases an enormous amount of heat. Yellowstone is hotter 7 kilometers down than almost anywhere else in the US. A large enough geothermal plant could tap this heat, generating electric power while at the same time reducing or eliminating the risk of a catastrophic eruption.
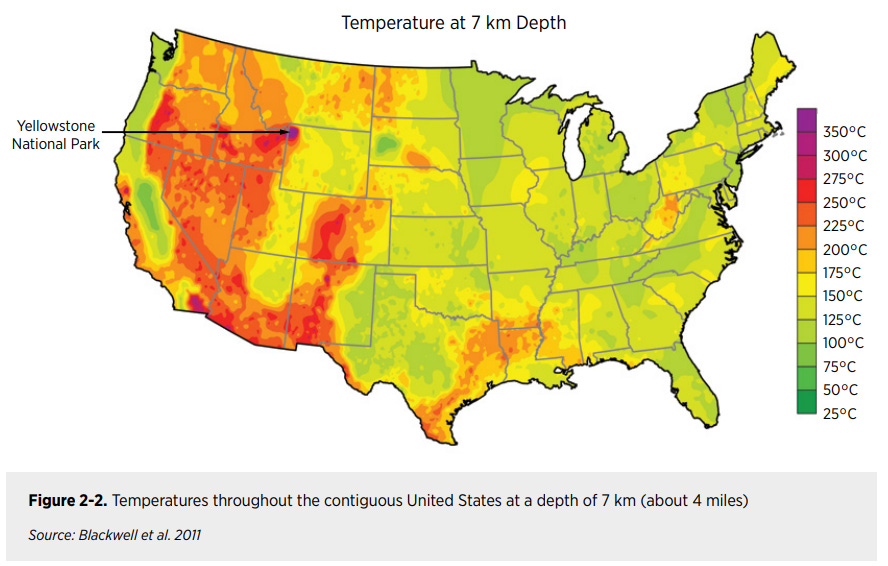
I’m aware of two proposals for doing this - one produced by NASA’s Jet Propulsion Laboratory in 2017, and a more recent one by Thomas Arciuolo and Miad Faezipour.
The JPL proposal
The JPL proposal is primarily aimed at reducing the risk of Yellowstone erupting, with electric power generation as a side benefit. The authors note that given the size of past Yellowstone eruptions, and the span of time between them, the rate that energy builds below the volcano is only around 1.5 gigawatts - less heat than a typical power plant sheds. Yellowstone currently bleeds heat at a rate of about 4.5 to 6 gigawatts, mostly through heated water moving below the surface. You'd thus (theoretically) only need to increase the heat bleed by around 35% to stop energy accumulating and stop future eruptions. [0]
Drilling into, or above, the magma chamber below Yellowstone would be fraught with problems. For one, it could potentially cause the volcano to erupt, the very thing we’re trying to avoid. For another, the environment above the magma body isn’t solid rock but is a “mush” of rock, acid, and brine that would be incredibly difficult to drill through. And even if you could successfully drill through it, the environment is so corrosive that even corrosion-resistant materials would start to corrode within days of exposure.
Instead, the authors suggest constructing a series of 160 geothermal plants around the perimeter of the magma body, spaced every 1.5 kilometers. Each plant would drill a pair of wells (a production well to draw water from, and an injection well to reinject it) up to 10 kilometers deep. At the projected flow rate of an enhanced geothermal system (80 kilograms a second), 160 plants together would extract about 20 gigawatts of heat energy, and generate around 3.5 gigawatts of electric power. At a projected cost of $1 per watt, such a system would cost on the order of $3.5 billion, and generate electric power for less than ten cents a kilowatt-hour, though I have been told that $1 per watt is an extremely optimistic projection for EGS cost.
This system would create a “ring” of cooled rock around the magma chamber around 50 meters wide. Every 50 years, a new series of plants would be constructed in a slightly smaller perimeter (or new wells would be directionally drilled from the existing plants), slowly growing the ring of cooled rock around the magma chamber. At this rate, the energy of the next eruption would be drained after about 16,000 years, and in less than 50,000 years the magma chamber would be cooled completely. While this is a long time, it’s short in the context of Yellowstone, which has only had three large eruptions in the past 2.1 million years.
3.5 gigawatts is less than the capacity of a single nuclear power plant. But the authors of the JPL paper note that “It is…straightforward to imagine that the entire capacity could be made several times the nominal 20 GW considered here, reducing proportionately the time to drain the heat from the magma chamber”. This is roughly what Arciuolo and Faezipour (which I’ll shorten to A&F) propose.
The Arciuolo and Faezipour Proposal
Conceptually, A&F’s proposal isn’t all that different from the JPL proposal, but it’s much larger in scale. In the A&F proposal, 100 shafts eight meters in diameter would be drilled eight kilometers down into the Yellowstone magma chamber. (By comparison, a typical geothermal well is around one meter in diameter at the top, and narrows as it goes down). A typical oil and gas well is eight to twelve inches in diameter). A large central pipe would extend to the bottom of the shaft, where it would split into ten smaller pipes and come back up, where it would feed into a large steam turbine. Water (the authors suggest using Shoshone Lake as a source) would be pumped down the central pipe, and it would come back up as superheated steam to drive the turbines.
A&F suggest gold-plated copper for the piping - gold-plating for corrosion resistance, and copper for heat conduction. Whereas the JPL proposal only extracted 20 gigawatts of heat from the ground, the A&F system is projected to extract more than 1200 gigawatts, over 60 times as much. Rather than taking 50,000 years to drain heat from the magma chamber, the A&F proposal would do it in around 830 years.
Other than the scale, the A&F proposal mostly differs from the JPL proposal in the details of the proposed energy extraction system. Unfortunately, as far as I can tell the A&F system is not especially well thought out, and most of the details provided don’t make much sense.
For one, the choice of gold-plated copper as a construction material is bizarre, and not appropriate for the harsh conditions of a geothermal well. Copper is comparatively soft and weak, and it loses its already low strength rapidly at elevated temperatures - not ideal for a geothermal well where temperatures are likely to exceed 1000 degrees Fahrenheit. Existing geothermal wells typically use steel or (for highly corrosive environments) titanium for the pipes and casing. The Iceland Deep Drilling Project, an experimental project which drilled a well into magma and generated power from the resulting steam, used K55 steel for the well casing.
Gold-plating the piping likewise doesn’t seem to make much sense. While gold-plating is often used for electrical connectors to provide corrosion resistance, the thin layer of gold wears away over time. Gold-plated jewelry wears through after a few years, and even mil-spec gold-plated connectors have a lifespan of a few thousand mating cycles (times being connected/reconnected). The environment in a geothermal well, where the piping would be exposed to high temperature water and steam flowing at thousands of liters a minute, and acid and brine-soaked rocks, is going to wear through a thin layer of gold plating much faster. It goes without saying that current geothermal wells do not use gold plated piping.
A&F calculated that their system would generate about 1260 gigawatts of electricity, more than the entire electric generation capacity of the US (1143 gigawatts as of 2021). But this calculation is incorrect. The authors assume that the conversion of heat to electricity would be roughly 90% efficient based on the efficiency of the turbine (minus a very small amount of energy needed to pump the water). But this 90% is the efficiency of the turbine, based on comparing it to an ideal, zero entropy turbine - it’s not the efficiency of the overall process. Any heat engine is fundamentally limited in how efficiently it can turn heat into useful work, and that limit is much lower than 90%. Existing geothermal plants operate at roughly 10-15% thermal efficiency. Coal plants which use supercritical steam can approach 50% thermal efficiency. The JPL proposal, which uses a similar temperature steam as the A&F proposal, calculated a 17% thermal efficiency. So A&F have overestimated how much electricity their system will generate by about a factor of five.
There’s also various other strange things about this proposal. A&F don’t seem to be using insulated pipes (in their analysis steam temperature falls from more than 1400 degrees F at the bottom of the shaft to around 600 degrees F as it travels back up from the magma chamber), even though with insulation losses should be on the order of a few percent (another reason why it doesn’t make sense to use copper). Their arrangement of the pipes (the downflow on the inside, and the upflow on the outside) is backwards - the water on its way down should be on the outside, to absorb heat from the surrounding rock. An eight-meter shaft (which would require a much larger diameter near the surface) would, as the authors note, require something akin to a tunnel-boring machine to drill, and would be vastly more expensive than using a larger number of smaller shafts.
Should we do it?
Regardless of the specifics of the details, in the abstract, such a scheme is in some sense, possible. How should we consider it?
On the one hand, building a huge geothermal power station at Yellowstone would generate a large amount of (potentially cheap) electric power while simultaneously reducing a catastrophic risk. Moreover, developing the technology to build it would have benefits that would extend beyond Yellowstone. Enhanced geothermal technology is still in the very early stages, and the advances required to build an enormous plant at Yellowstone could profitably be applied to other plants. Likewise, the knowledge gained figuring out how to stop one supervolcano from erupting could be applied to preventing other supervolcanoes from erupting. And more broadly, stopping supervolcanoes from erupting is something we as a civilization are likely underinvesting in. In “The Precipice'', Toby Ord estimates that the risk to civilization from supervolcano eruption is 100 times greater than the risk from asteroid and comet impact, which NASA spends over $100 million on each year. Based on that, we should be spending at least on the order of $10 billion every year on preventing supervolcano eruptions.
On the other hand, in many ways Yellowstone is a particularly bad place to try to build such a plant. The harsh, corrosive conditions in and around the magma chamber would make drilling the wells especially difficult, and its location in the middle of nowhere would require the construction of enormous transmission lines (something the US is not so great at). One of the main benefits of enhanced geothermal is that you can construct it nearly anywhere, enabling things like using geothermal directly for industrial process heat (eliminating wasteful conversions of heat to electricity to heat again), or repowering existing coal plants (reusing their steam turbines and other electrical infrastructure). A massive Yellowstone geothermal plant gives up a lot of these benefits.
And while Yellowstone is at risk of a catastrophic super-eruption, it’s extensively monitored by the Yellowstone Volcano Observatory. We’d (hopefully!) have advanced warning long before Yellowstone actually erupted. A much bigger risk is likely large eruptions from volcanoes that we aren’t tracking and have no data for. Data from ice cores suggests there have been 97 large-magnitude volcano eruptions in the last 60,000 years, but only a handful of those can be attributed to specific volcanoes. Of all the volcano eruptions that have occurred since 1950, only 27% were monitored by any sort of instrument such as a seismometer prior to eruption. There are no dedicated satellites for monitoring potential volcano eruptions.
In any case, the debate is likely to remain academic for the foreseeable future. Using Yellowstone for geothermal power was made illegal by the Geothermal Steam Act of 1970, which “requires the Department of the Interior to preserve and monitor hydrothermal features like Old Faithful”. And while there’s increasing realization that extensive environmental review can often harm the environment, and that some kind of permitting reform will be necessary to build out low-carbon energy infrastructure, we’re still in the early days. Trying to build an enormous geothermal power plant and associated transmission lines(!) in one of the most beloved National Parks(!!), which there’s specifically a law against (!!!), and which could potentially trigger a civilization-destroying volcanic eruption (!!!!) is like the final boss of the permitting reform movement. [1]
(Thanks to Austin Vernon for reading a draft of this. All errors are my own!)
[0] - If instead you look at the rate that magma is accumulating in the lower magma chamber, you get a larger, but still manageable number of around 22 gigawatts.
[1] - The only thing it's missing is rich NIMBYs who would be inconvenienced by the construction.
I struggle to believe that someone who proposes gold plated copper for a subterranean pipe has an idea what they’re talking about
I mean the Dutton ranch is close to Yellowstone, so the rich NIMBYS will be there for sure