(Note: unless noted otherwise, quotes are from John Perlin’s “From space to earth: the story of solar electricity”)
Solar photovoltaics (PV) have become one of the cheapest sources of electricity. Lazard’s estimate of unsubsidized levelized cost of energy (LCOE), the average cost of electricity generated over a plant’s lifetime, has utility scale solar PV cheaper than anything except completely depreciated natural gas plants and wind in the very windiest locations. Data from the Energy Information Administration (EIA) likewise shows that new solar PV plants in the US generate electricity cheaper than anything else except wind power in the best locations.
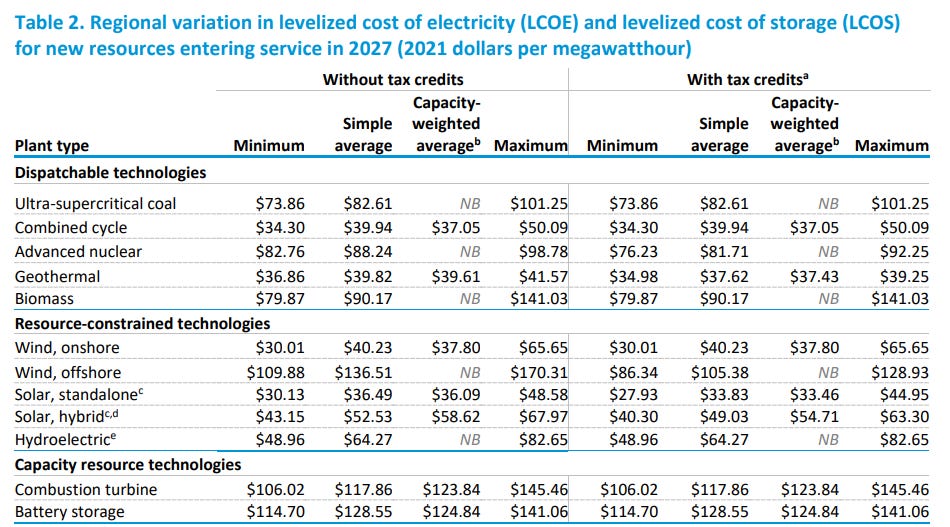
Solar PV’s low cost is the result of it steadily falling in price over many decades. In 1957 solar PV electricity cost roughly $300,000 per megawatt-hour in 2019 dollars. By 2019, in the sunniest locations that had fallen to roughly $20 per megawatt-hour, 15,000 times less. And it's still getting cheaper. In 2021, the DOE set a goal to reduce the cost of solar PV by another 50% by 2030.
Because of its low cost, while solar PV is still a small fraction of overall electricity generation (around 6% in the US), it’s an increasingly large fraction of new electricity generation capacity. Of the 151 gigawatts of planned electricity generating plants tracked by the EIA, 49% of them are solar PV projects.
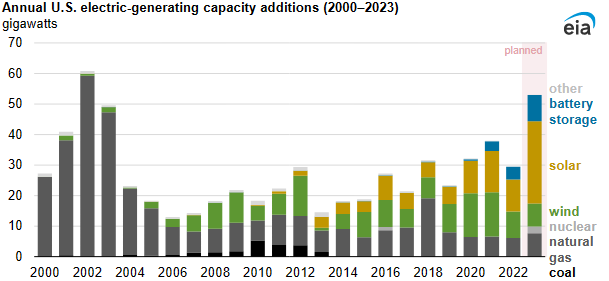
Similar trends are happening worldwide. Globally, installed solar PV capacity is increasing by roughly 20% to 30% per year. Worldwide solar PV generation went from 34 terawatt-hours in 2010 (around 0.2% of total electricity use) to over 1000 terawatt-hours in 2021, close to 5% of world capacity.
How did this happen? Why did solar PV electricity get so cheap? Let’s take a look.
Origin of solar photovoltaics
Solar PV works via the photovoltaic effect (a cousin of the photoelectric effect) [0], where electrons are excited when electromagnetic radiation (such as light) strikes a material. The earliest exploration of the photovoltaic effect dates to 1839, when Edmond Becquerel (father of Henri Becquerel, discoverer of radioactivity), created an electric current by placing silver chloride in acid and exposing it to light.
The first use of the photovoltaic effect to generate electricity took place roughly 40 years later. In the 1860s, Willoughby Smith was searching for a material to use in a device for finding flaws in submarine telegraph cables. One of the materials tested, crystalline selenium bars, was found to work well at night, but failed mysteriously during the day. Smith experimented with the bars and found that exposing them to light greatly increased their electrical conductivity. Smith published his findings in 1873. Further experiments on crystalline selenium by William Adams and Richard Day revealed that the selenium could actually produce an electric current when exposed to light, which they called “photoelectric current”.
In 1881, American inventor Charles Fritts used this discovery to build the world’s first solar PV cell. Fritt’s cell consisted of a thin layer of selenium on top of a copper plate, covered with semitransparent gold leaf. Fritts found that when exposed to light, his cell could produce a current “that is continuous, constant, and of considerable force”.
Fritts showed his invention to famous inventor and industrialist Werner von Siemens, who encouraged other scientists to explore the potential of solar generated electricity. But Fritts’ selenium solar panels were incredibly inefficient, converting less than 1% of the light’s energy into electricity. Development of light-sensitive selenium panels continued (they later became popular as light meters for cameras), but selenium PV cells were never widely used as a source of electricity. In 1935 the head of Westinghouse’s photoelectricity division stated that “as a power converter the photovoltaic cell will not even prove interesting to the practical engineer until the efficiency has been increased at least 50 times.”
Invention of the modern solar cell
The modern solar PV cell was invented at Bell Labs in 1954, more than 70 years after Fritts built his selenium solar cell. In 1940, while experimenting with silicon crystals for radio transmission, Bell Labs engineer Russell Ohl noticed a silicon rod with a crack in it produced strange electrical behavior, including a surprisingly strong flow of electricity when exposed to light. Taking samples of the silicon, Ohl and his staff found that there were slight chemical differences on each side of the crack. The silicon rods had been cut from silicon ingots that were made in a furnace, and the ingot Ohl’s rod had been cut from had been cooled very slowly when taken out of the furnace to try and prevent cracking. As the ingot cooled, chemical impurities in the silicon had migrated - lighter impurities migrated to the top, while heavier impurities remained at the bottom. These different impurities were causing the photovoltaic phenomenon at the crack which separated the two regions. By chance, Ohl had stumbled across a p-n junction, a fundamental building block of semiconductor technology and the heart of modern solar cells.
Several years later, another Bell Labs scientist, Darryl Chapin, was looking for a way to power remote telephone repeating stations in the tropics. Chapin looked at several possible power sources, including “wind machines, thermoelectric generators, and small steam engines”, as well as selenium solar PV cells. After the selenium cells were found to be just 0.5% efficient at converting sunlight to electricity, Gerald Pearson, a colleague working with silicon p-n junctions in transistor research, suggested that Chapin replace the selenium cells with silicon ones. The silicon PV cells proved to be 2.3% efficient at converting sunlight to electricity, more than four times as efficient as the selenium cells. Chapin, Pearson, and Calvin Fuller (another Bell Labs scientist) further improved the silicon cells by adding an antireflective coating, changing the orientation of the p-n layers, and finding ways to make the junction just 0.0001 inches below the surface (letting more light reach it). By 1954 (the year construction started on the first civilian nuclear reactor at Shippingport), Chapin’s best silicon PV cells had reached 6% efficiency.
Bell Labs announced their “Solar Battery” in a press conference in April of 1954, to great fanfare. The announcement made the front page of the New York Times [1], and an article in US News and World Report speculated that “The [silicon] strips may provide more power than all the world’s coal, oil, and uranium.”
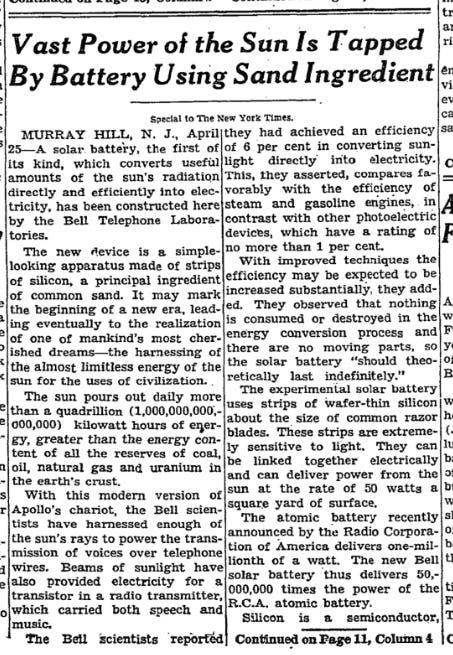
How a solar PV cell works
Today’s solar PV cells work on essentially the same principle as the ones developed at Bell Labs in 1954. Briefly, an atom consists of a positively charged nucleus surrounded by a negatively charged cloud of electrons. This electron cloud is divided into “shells”, which can each hold a certain number of electrons. Atoms tend to be stable when their outermost electron shell (called the “valence shell”) is completely filled. Atoms can fill this shell by sharing or transferring electrons in it (“valence electrons”) with other atoms. Hydrogen, for instance, has just one electron in a shell that can hold two electrons. If two hydrogen atoms share their electrons (forming an H2 molecule, the naturally occurring form of hydrogen), they can each have a filled valence shell.
Silicon, which has four electrons in its valence shell, will naturally bond with four other silicon atoms, sharing one electron with each. However, if we replace a small number of silicon atoms with atoms that only have three valence electrons [2], like boron, this will create electron “holes” - places that could be occupied by valence electrons but aren’t. Electron holes can be thought of as positively charged particles (since they’re the absence of a negatively charged electron). Silicon that has a small amount of boron or other impurities with only three valence electrons is known as p-type silicon (for positive).
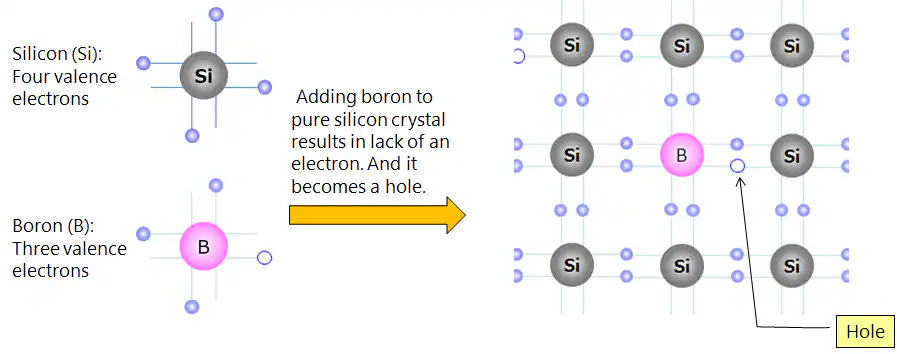
Likewise, if we substitute a small number of silicon atoms with atoms that have five valence electrons, such as phosphorus, this will create an “extra” electron. This is known as n-type silicon (for negative). (Note that both p and n-type silicon remain electrically neutral, since the additional electron/hole is balanced by the appropriate number of protons in the nucleus).
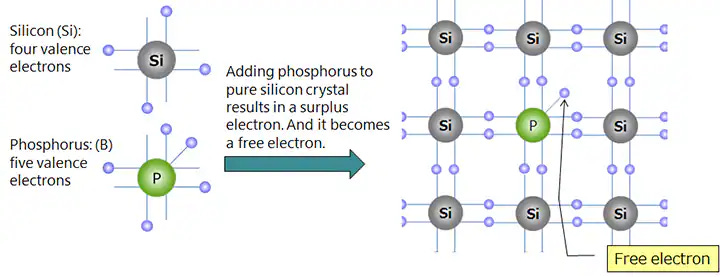
If we put a layer of p-type silicon next to a layer of n-type silicon, diffusion will cause the free electrons and electron holes to migrate - free electrons will head to the p-side and electron holes will head to the n-side. The area where electrons and holes have migrated is called the 'depleted area'. Within the depleted area, the imbalance of charges (negatively charged electrons migrating to the formerly neutral p-region, and positively charged holes migrating to the formerly neutral n-region) creates an electric field, which stops further diffusion.
When light strikes the depleted area of a p-n junction, it can excite an electron (kicking it from the valence band into the conduction band). This excited electron leaves behind an electron hole. Because of the electric field in the depleted area, the negatively charged electron gets pushed to the n-side of the junction, and the positively charged hole gets pushed to a p-side. This creates an electric potential on each side of the cell - free electrons collecting on one side, and electron holes on the other. Connect the two sides, and electrical current will flow as the charges try to reestablish equilibrium [3].
Early use of solar cells
Like with many new technologies, enthusiasm had outrun solar’s actual capabilities. Making a silicon PV cell required extremely high purity silicon, which at the time cost $380 a pound ($4200 a pound in 2023 dollars). The expense of early solar PV cells made them impractical for most potential uses. In 1956 Chapin calculated that powering the average home with solar PV cells would cost nearly $1.5 million. Companies that had licensed Bell’s silicon PV technology pitched it to the Coast Guard and the Forest Service as a source of remote power without success - solar PV electricity was more than five times as expensive as batteries, and 10,000 times as expensive as electricity from utilities. Using solar PV in toys, radios, and hearing aids (the cells were mounted to eyeglasses, though none were ever produced) likewise proved to be a flop. Chapin began to wonder “What to do with our new baby?”
But while manufacturers tried unsuccessfully to sell solar powered radios, the industry that would be the main customer for solar PV for the next 20 years was being created. In October 1957, the Soviet Union launched the world’s first artificial satellite, Sputnik-1, kicking off the space race between the US and the Soviet Union. The US launched its own satellite, Explorer-1, several months later. Both Sputnik-1 and Explorer-1 were battery powered, but batteries were far from ideal as a satellite power source. Batteries were heavy (40% of the weight of Explorer-1 was its batteries) and could only provide power for a short time. The batteries on Sputnik I lasted just 21 days. On Explorer-1, they lasted four months. Not only were solar PV cells much lighter than batteries, but they could potentially power a satellite for years.
The US Army Signal Corps had visited Bell Labs to explore the potential of solar PV cells on satellites as early as 1955. The first solar powered satellite, the Vanguard I, was launched by the US in March of 1958, just three months after Explorer-1. Where Explorer-1 had run out of power after four months, Vanguard’s solar cells kept it operational for 6 years. Three weeks after Vanguard, the Soviet Union launched Sputnik III, also powered by solar PV cells. By 1972, the US and the Soviet Union together had launched more than 1000 satellites, nearly all of them powered by solar PV. Of the 600 American satellites in orbit in 1972, all but 10 of them used solar PV cells. Not only could solar PV cells supply power for long periods of time, but they could operate at any required scale, from milliwatts of power on Vanguard I to kilowatts (millions of times more) on Skylab. Solar PV became “one of the critically important devices in the space program”, as it “turned out to provide the only practical power source in space [at a reasonable distance to] the sun”. Between 1958 and 1969, $50 million (nearly $500 million in 2023 dollars) was invested by the US government in solar PV development for satellite use. As late as 1974, satellites made up 94% of the market for solar PV.
As the market for solar PV expanded, the cost of it fell. Between 1956 and 1971, the cost of solar PV electricity dropped 300 percent, to $100 per watt. However, this was still far more expensive than other sources of electricity. To be useful outside of the space industry, solar PV would need to get even cheaper.
This was accomplished, perhaps ironically, with the help of the oil and gas industry. In 1969, an industrial chemist, Elliot Berman, formed Solar Power Corporation with the goal of providing the world with power using solar energy. Berman felt that “in the long term…only nuclear fusion would meet [future energy] needs and this simple-minded chemist chose the easier course, using our existing fusion plant - the sun.” Berman’s pitch was rejected by venture capitalists, but he was able to secure funding from Exxon, who had projected future global energy demand and felt there would be opportunity for alternative energy sources such as solar. (Exxon wasn’t alone - nearly every major oil and gas company “invested heavily in solar cells, buying controlling interests in many fledgling photovoltaics companies”).
Building to the requirements of spaceflight had forced solar PV to make expensive choices. Each cell was custom designed for the satellite it would be attached to. Because weight was at a premium, cell efficiency had to be maximized, which meant using extremely pure (and extremely expensive) silicon. To pack the cells as tightly together as possible (and because the edges often had defects), round silicon wafers were cut into rectangles (wasting the rounded silicon at the edge). And because servicing or replacing satellite PV cells in orbit was impractical, they also needed to be extremely reliable, with a lifespan of 20 years or more.
But PV cells that would be used on earth didn’t require all this. Solar Power Corporation was able to cut costs significantly by using waste silicon wafers from the computer industry - the defects that made the wafers unusable for chips were acceptable in a solar PV cell. Solar Power Corporation also used wafers more efficiently by using the entire wafer, rather than cutting off the rounded edges. And rather than polishing the wafers and then adding a nonreflecting coating, Solar Power Corporation simply used the unpolished wafers, which naturally had a dull, non reflective surface, cutting out two expensive manufacturing steps. The result of these and other changes was a less efficient, less reliable, but much cheaper solar PV cell. By 1973 Solar Power Corporation was able to produce solar PV electricity for $10 per watt, and sell it for $20.
In addition to funding many early photovoltaic companies, oil and gas companies became some of the first major terrestrial users of these cheaper solar PV cells. Prior to solar PV, offshore drilling platforms often required batteries to provide power, which were expensive, bulky, and required frequent maintenance. Solar PV cells proved much cheaper and easier to maintain. By the late 1970s, hundreds of solar PV modules had been sold to nearly every major oil and gas company for use on offshore oil platforms. Oil and gas companies also used solar PV on land, to power cathodic protection systems on remote oil wells to prevent them from corroding.
Outside of oil and gas, solar PV was becoming an increasingly popular choice to provide power for remote locations. Between 1961 and 1972, Sharp installed 226 solar PV systems on Japanese lighthouses, and in 1973 the US Coast Guard began to use solar PV to power lights on navigational buoys (replacing acetylene gas lanterns). Greece and France likewise converted their navigational buoys to solar PV in the early 1980s. In the late 1970s and early 1980s, Telecom Australia became a major purchaser of solar PV modules to power remote telephone repeaters. By 1979, terrestrial uses had grown to 64% of the solar PV market, up from 6% in 1974.
Government funding of solar PV
Development of solar PV was also, like every energy technology, accelerated by the 1970s energy crisis. After OPEC cut oil production in 1973 in response to the US supporting Israel in the Yom Kippur War, the price of oil rose by a factor of four. One month later, President Nixon announced “Project Independence”, an initiative to make the US energy independent by 1980. Though focus was chiefly on development of coal, nuclear, and synthetic fuels, there was also increased focus on solar PV. In 1977 the government created the Solar Energy Research Institute (SERI), which later became the National Renewable Energy Lab (NREL), and the federal government poured $1.7 billion dollars into solar PV research between 1974 and 1981.
The most important government solar PV initiative during this era was likely the series of “Block Buys” by the Energy Research and Development Administration (ERDA) (which would later become the Department of Energy). In this program, ERDA agreed to purchase a certain amount of solar PV cells from private companies, at specific prices and performance targets. The purchases were done in a series of five “blocks”, with each block having more stringent performance requirements. Most US solar PV companies, including Solar Power Corporation, participated, and “almost everyone in the PV community” was a partner in the program in one way or another. Between 1976 and 1981 the Block Buy program purchased 400 kilowatts of solar PV modules, about 17% of the total PV market at the time. (Today, annual solar module production is 268 gigawatts, or about 570,000 times as large as the market in the late 1970s).
The cost of solar PV continued to fall. Silicon got cheaper, PV plants got larger and saw greater economies of scale, and PV modules got more efficient. By the early 1980s, the cost of solar PV had fallen to $11 per watt, and solar PV had grown from a niche supplier of satellite components into an industry in its own right. Solar PV sales by US companies went from $7 million in 1976 to $100 million in 1982. By 1981, 4,000 people were working in solar PV.
Up through the early 1980s, the US had been the leader in the development and manufacturing of solar PV. But this would come to an end. The second energy crisis of 1979 was followed by a collapse in the price of oil in the mid-1980s. And though solar PV had gotten much cheaper, it was still far from being competitive with utility scale electricity. Federal funding for solar R&D was cut by the Reagan administration, and the Block Buy program halted. Exxon closed Solar Power Corporation in 1984. The mantle of solar PV development would be taken up abroad, by Japan, Germany, and finally China.
This series will conclude next week with Part II
Sources
Gregory Nemet - How solar energy became cheap
Gregory Nemet - Beyond the learning curve: factors influencing cost reductions in photovoltaics
Kavlak et al - Evaluating the causes of cost reduction in photovoltaic modules
John Perlin - From space to earth: the story of solar electricity
Michael Riordan - Crystal fire: the invention of the transistor and the birth of the information age
Martin Green - Silicon Photovoltaic Modules: A Brief History of the First 50 Years
Footnotes
[0] - Today, the term photoelectric effect is used when electrons are ejected from a material, whereas the photovoltaic effect is when an electron is excited to a higher energy state but remains in the material.
[1] - By comparison, Bell Labs’ announcement of the transistor only made page 46 of the New York Times.
[2] - On the order of replacing one out of every million silicon atoms.
[3] - This is a very simplified explanation that leaves out lots of critical parts. For a more in depth explanation, I might try this multi-part video series on Khan Academy.
In paragraph 2 below the chart, should “megawatt” be “megawatt-hour”?
One quibble:
"As the market for solar PV expanded, the cost of it fell. Between 1956 and 1971, the cost of solar PV electricity dropped 300 percent, to $100 per watt."
No, it didn't. Costs can rarely fall more than 100 percent because anything falling 100% ends up at a cost of 0. Didn't see the numbers behind this claim, but I'm guessing it fell much less.