(See here for Part I)
When we left off in the 1960s, what few wind power efforts that existed had largely stalled. Commercial manufacturers that supplied wind power to rural customers disappeared as electrical grids and transmission lines were extended to reach them, and research efforts were cancelled or scaled back as conventional electricity prices continued to fall. Focus was placed on nuclear power as the next generation of electricity technology.
However, two events would change the calculus for wind power.
The first was the rising environmental movement. As concern grew over the impact humans were having on the environment, power-generating technologies like wind power, which burned no fuel, emitted no pollutants, and required no radioactive substances, became much more appealing. And the passage of environmental legislation in the late 1960s and the early 1970s, such as the Clean Air Act and Clean Water Act, made other, higher-impact sources of power more expensive. After 1970, the cost of a new coal plant (which at the time generated 45% of the US's electricity), which had been dropping steadily for decades, began to rise.
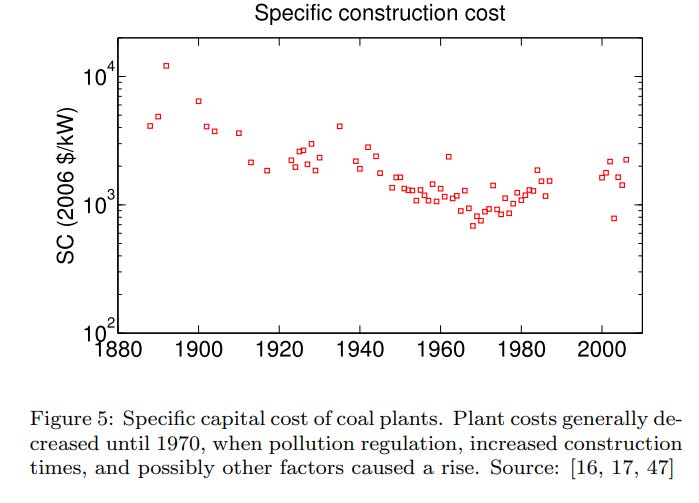
But the more immediate effect was from the 1973 Energy Crisis. In response to the US's support of Israel in the Yom Kippur War, the Organization of Petroleum Exporting Countries (OPEC) instituted an oil embargo in the fall of 1973, causing oil prices to jump from $3 per barrel to $12 per barrel. Securing an energy supply became a national priority for countries around the world.
Prior to the embargo, the Nixon administration had commissioned a report from the commissioner of the AEC to evaluate the country's energy supply. That report, "The Nation's Energy Future", recommended a multi-billion-dollar government investment in energy generation technologies to achieve energy self-sufficiency, including investments in wind power research. At the time, the only government-funded wind power research in the US was a small project being led by NASA (which was searching for new purpose following post-Apollo budget cutbacks), the Federal Wind Energy Program (FWEP). FWEP would become the main vehicle for US government wind energy R&D, receiving $1.8 million in funding in 1974, rising to $60 million in 1980.
Government wind R&D
Until that point, most commercially successful wind turbines had largely been small-scale, used to generate electricity for individual rural households or small communities. Most Jacob wind turbines, for instance, were in the range of 1-3 kW, and the largest Dunlite turbine was only 5 kW. Allgair's Hutter-designed turbine sold in the 1950s was 10 kW. By comparison, a large nuclear power plant might have a capacity of 1000 MW, or 100,000 times as much as a Hutter turbine.
NASA's aim was to quickly develop large, 1MW+ turbines that could be used for large-scale power generation. It held a series of workshops with wind power experts throughout the 1970s, and funded the rebuilding of Juul's Gedser turbine (which had been sitting in storage) for testing. NASA's first test turbine, the 100 kW MOD-0, was built at at Plum Brook Station near Cleveland in 1975. The MOD-0 was based on the designs of Ulrich Hutter and operated as NASA's testbed for 10 years.
The MOD-0 was followed by a series of successively larger turbines:
4 200 kW MOD-0A turbines were built between 1977 and 1980 in Puerto Rico, New Mexico, Rhode Island, and Hawaii (one by NASA and 3 by Westinghouse). Westinghouse later developed and sold a larger, 600 kW
A 2 MW MOD-1 was built by General Electric in 1979.
Three 2.5 MW MOD-2 turbines were built in 1982 in Washington State by Boeing.
A 3.2 MW MOD-5B built in 1987 in Hawaii, also by Boeing.
These turbines largely failed to live up to expectations. The MOD-1, intended to be the first economically competitive large-scale turbine, produced electricity at the uncompetitive rate of 20 cents per kWh, compared to the average electricity price in 1979 of 5 cents per kWh. It operated for just 130 hours before an expensive shaft failure caused it to be mothballed and eventually sold for scrap. Likewise, the MOD-2 turbines exhibited higher-than-anticipated stresses, and stopped operating in 1986 (and were likewise sold for scrap). The MOD-5B was sold to a power utility and operated intermittently until 1996, when it was also sold for scrap. Several other planned MOD turbines were cancelled. None of the NASA designs were successfully commercialized, and GE, Westinghouse, and Boeing all left the wind turbine market (GE would eventually return after acquiring Enron's wind-power division in 2002 following the company's bankruptcy).
Outside of the US, other government-led R&D programs to develop large-scale turbines mostly fared similarly. The most notable of these was the German Growian turbine, a large 3MW turbine designed by Ulrich Hutter and built in 1983. It experienced numerous mechanical problems, operating for only 420 hours before being dismantled in 1988. Similar large turbine research projects in Sweden, the UK, Italy, the Netherlands, and Denmark also failed to result in commercially successful turbines.
However, the technology used on NASA and other government R&D turbines would ultimately be widely deployed on a commercial basis. Things such as variable speed turbines, lightweight tubular towers, fiberglass turbine blades, Viterna methods for calculating turbine performance, and multimegawatt turbines were all used on NASA's turbines and all adopted by the industry eventually - the research efforts simply tried to move too fast towards commercialization. As stated in “The Wind Power Story”
In hindsight, it is more accurate to say that while NASA wasn’t necessarily moving in the wrong direction, they were trying to get there too quickly. As wind turbine technology history demonstrates, you cannot simply upsize from a 100‐kW to a 1‐MW wind turbine and maintain acceptable levels of reliability and performance. Instead, wind researchers—both public and private—would need to build and test successively larger wind turbines over a period of decades—not years—to reach the 1‐MW level in the late 1990s.
Small wind
That scaling would start with the small wind turbines which were once again being designed by a variety of wind power enthusiasts and entrepreneurs.
Many of these offerings were located in Denmark, which had both a long history of wind power and an urgent need for alternative energy sources - in 1970, 94% of Denmark's energy came from imported oil, with the rest coming from imported coal. Following the oil embargo and the oil price spike, Denmark also began to look towards energy self-sufficiency.
Though it was not initially a focus of the government (the country's national energy plan, published in 1976, made no mention of wind or solar, instead focusing on coal, natural gas, and nuclear power), Danish individuals and companies began to develop their own small wind turbines. Unlike the US R&D turbines, which were mostly developed and built by aerospace companies, the smaller Danish turbines were designed and built by carpenters, blacksmiths, farm equipment manufacturers, and students. Christian Riisager, for instance, was a Danish carpenter who successfully sold small wind turbines of his own design until 1985.
The most successful of these efforts was Vestas, which at the time was a manufacturer of cranes, farm equipment, and household appliances. In the 1970s the Danish farm equipment market was struggling, and Vestas, looking to enter the alternative energy market, introduced a small (30 kW) wind turbine on the market in 1979, following it up with a larger 55 kW model in 1981.
These Danish efforts were buoyed by government incentives passed in 1979 that provided a subsidy for renewable energy investments, and guaranteed loans for manufacturers who exported renewable energy equipment. By 1980, there were 10 registered firms selling wind turbines in Denmark.
There were similar small-scale efforts in the US. The Department of Energy created a wind-turbine test site at Rocky Flats in the mid 1970s, and from 1976 to 1982 54 different turbines from a variety of manufacturers were tested there. In 1974 the American Wind Energy Association (AWEA) was formed, and by 1979 had 1200 members.
The California wind rush
NASA's research projects weren't the only US government efforts to try to advance wind power. In 1978 the National Energy Act was passed, which added a 10% investment tax credit to an existing 10% credit (for a total of 20%) for alternative energy projects, which rose to 25% in 1980. It also included provisions which required utilities to purchase wind-generated (and other renewable) electricity that was supplied to the grid at favorable rates, in effect allowing anyone to build a wind turbine and starting selling their electricity.
On top of these federal incentives, the State of California passed its own 25% tax credit for wind power in 1979. Combined with federal incentives, investors could earn a nearly guaranteed profit by investing in wind power projects. From "Reaping the Wind"
What these tax credits did was make a wind turbine investment analogous to a real estate deal...The capital account that served as the basis for the tax write-off included not only what the investor had sunk into the project but also what the lender had loaned the investor...A common deal was a $25,000 investment in a $100,000 share of a wind turbine...What the 25 percent tax credit translated into for the investor was an immediate $25,000 tax break. In addition, substantial tax breaks, totaling up to 75 to 90 percent of the original loan amount, would persist over seven years as depreciation was exhausted. "In essence, the investor got all of his profits for nothing," Duskin said..."The tax breaks made wind very attractive. The investor got a lot of potential value for basically zero risk.”
(Another key California incentive was the “Interim Standard Offer”, which specified the rates that utility providers would pay for wind power. These were based on expected avoided costs of power, and were fixed for 10 years, which ended up providing a massive boon for wind power investors when oil prices fell in the late 1980s).
Thanks to these generous incentives, beginning in 1980 California saw a massive investment in wind power. By 1986 96% of the world's wind-generated electricity was being generated in California, and by 1987 California had nearly 17,000 wind turbines producing more than 1.2 GW of electricity. Unlike previous wind turbines (which were typically stood alone or in small groups), these turbines were mostly in large "farms" of many turbines, built by companies like US Wind, Zond, and Seawest.
Many of these were built by US companies - US Wind designed and built its own turbines, and other providers purchased them from companies like Enertech. But the boom was also a boom for Danish wind turbine manufacturers, who for the first time had a large market to sell into. The Danish-made turbines were sturdier more reliable than the American made turbines (though all manufacturers had to improve their designs to cope with the harsh California climate), and by 1987 other than turbines produced by US Wind, all turbines being installed in California were Danish. In 1992 43% of the wind turbines in California were from Denmark, and between 1979 and 1986 Vestas grew from 200 employees to 870 employees.
But the tide turned for the California wind experiment. The energy crises of 1973 and 1979 gave way to an oil glut in 1986 - oil prices fell from $35 per barrel in 1980 to $10 per barrel in 1986. The Reagan administration was less inclined to spend government funds on wind power development, and the wind power tax credits were increasingly seen as a tax dodge for wealthy investors (Forbes would later call it "The Great Windmill Tax Dodge"). Both federal and California credits were removed or allowed to expire, and turbine installation in the US collapsed:
The wind energy industry struggled following the collapse of the California wind market. Many manufacturers, including Vestas, declared bankruptcy (Vestas after a customer failed to pay for 1200 wind turbines that had been delivered).
European expansion
However, as the US market was declining, the European market was rising, also largely due to government incentive programs. In 1990 Germany passed a Feed-in-Tariff, which required utilities to purchase wind power at favorable rates, which became a popular mechanism in other European countries. Denmark's parliament agreed to develop 100 MW of wind power between 1986 and 1990, and passed its own Feed-in Tariff in 1990. The UK similarly passed a Non-Fossil Fuel Obligation (NFFO) program in 1990, and by 1995 had installed 250 MW of wind power. By 2000, more than 70% of the world’s wind energy was being generated in Europe, mostly in Germany, Denmark, and Spain.
The US market was largely flat in the 1990s, but returned as individual states began to pass Renewable Portfolio Standards, which mandated some fraction of their electricity be produced via renewable sources such as solar and wind. (Texas’s portfolio standard was passed partly thanks to lobbying by Enron, and was championed by then-governor George W. Bush). In addition, a production tax credit, which provided a tax credit for each kW of wind power generated, was passed in the early 90s and remains in place today (though it has been allowed to expire several times). This policy infrastructure - renewable portfolio standards and a production tax credit in the US, Feed-in-Tariffs in Europe - is largely the policy infrastructure that exists for wind power today.
And as more and more wind turbines were built, the cost of wind-generated electricity steadily fell, approximately 74% from 1984 to 2014, and another 32% between 2014 and 2019.
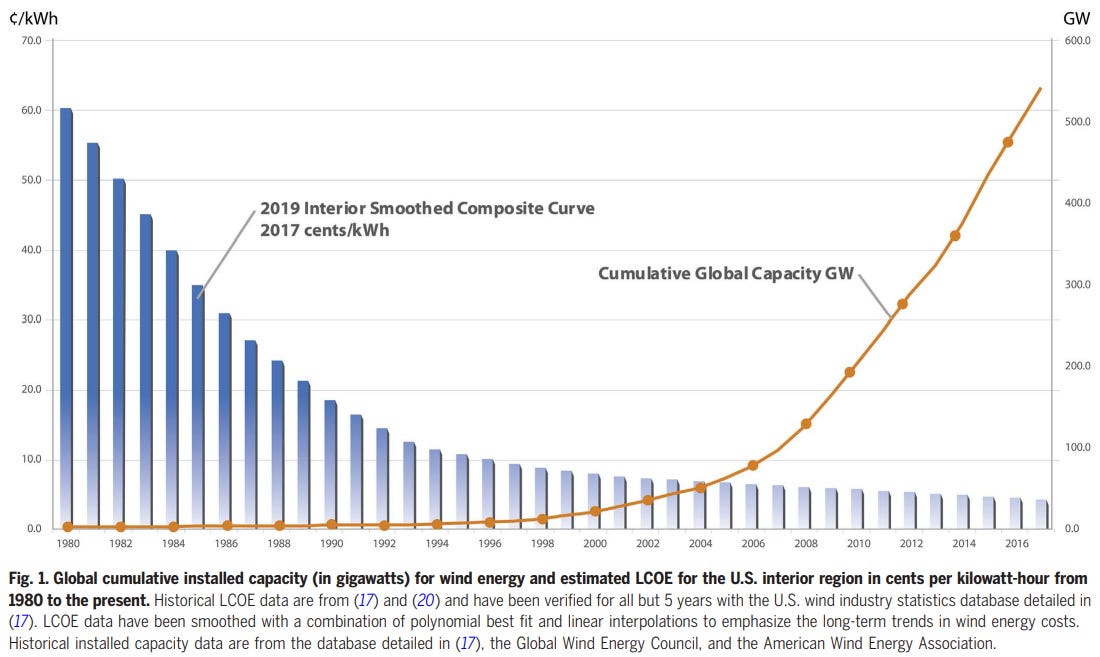
Sources of cost reduction
After more than a century of being an uncompetitive and expensive source of electricity, wind power has finally gotten cheap - the incentives, tax credits, and other policy measures encouraged enough turbines to be built that manufactures figured out how to make them more and more efficiently. What sort of improvements were at work?
Partly this was a result of improved reliability. By the 1990s the industry had converged on a design (3-bladed, upwind turbines on a steel monopole) that minimized component fatigue and problems of excess vibration. By 2000, capacity factors for turbines were between 25 and 30 percent, twice the rate of the failure-prone turbines built in California in the 1980s. Not only does this mean the turbine is operating a larger fraction of the time, but it also means maintenance costs are lower.
There were other technology improvements as well. Most early grid-connected turbines, for instance, rotated at a constant speed to more easily synchronize with the AC electrical grid. There were eventually superseded by more efficient, variable-speed turbines. Efficiency was also improved by better modeling and understanding of the wind resources available at a given site. And as more wind projects were built and they became better understood, the project risk was reduced and financing costs dropped.
But in large part, the decreasing cost of wind power is a story of increasing windmill size. The early turbines of the 1980s were largely 50-100 kW in power, and stood 50-60 feet tall. Over time, this has increased - the average onshore turbine height today is well over 300 feet, with a capacity of 3 MW or more.
There’s a variety of economies available to larger turbines. A taller wind turbine has access to higher and steadier winds that are less affected by friction against the ground - because wind energy rises with the cube of windspeed, even a small increase in speed can have a large impact on energy generated. Fewer, larger turbines means fewer moving parts, fewer additional costs from things like transmission cables. Longer windmill blades capture more of the surrounding energy, and (if generator rating remains constant) allow the turbine to more frequently operate at close to full output. And unlike construction costs for buildings, the cost of constructing a turbine doesn't (as I understand it) rise linearly with turbine size - even a 3 MW turbine can often be erected in a single day - making larger turbines cheaper to construct on a cost-per-watt basis.
However, there are also diseconomies at work with increasing turbine size. A larger, taller turbine requires a larger crane to erect, and requires more work to be done in the field rather than the controlled conditions of a factory. The higher wind speeds mean higher lateral forces, and bending moments in the support tower and the windmill blades will rise with the square of height/length. And while available wind energy will rise with the square of rotor length, the mass of the rotor will (all else being equal) rise with the cube of rotor length.
Similarly, increasing use of wind power has negative economies with respect to site selection - as the windiest sites get built out, turbines must increasingly be built at locations with more marginal wind resources.
This tension in scale effects in wind turbine size has historically resulted in disagreements between experts over what the optimal size of a turbine is. In the mid 1990s many experts argued that it was uneconomical for turbines to grow beyond a certain size. Paul Gipe (a small wind proponent) notes some of these arguments in his 1995 "Wind Energy Comes of Age":
Wind turbines are like heat exchangers, Jamieson contents. They require lots of surface area to perform their task productively. Thus, he says, it is better to have lots of small machines than to have one big one, just as it is better to have lots of small fins than to have one big one on a radiator. There is "less mass in a multiplicity of structures" intercepting the same area. There is also an economic advantage from the improved reliability because of the redundancy of multiple machines, regardless of their greater infrastructure cost for more wiring and transformers.
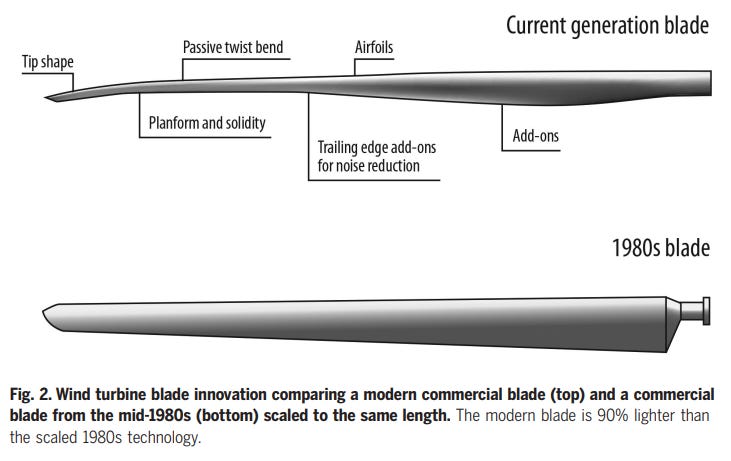
The average turbine capacity has increased almost 10x since that was written. The continued increase of wind turbine size has required finding ways around these negative scaling effects. A modern wind turbine blade, for instance, weighs about 90% less than an equivalent length blade from the 1980s, and the actual rotor weight scaling exponent with respect to length has been closer to 2.2 than to 3. Increases in the size of rotor for a given turbine rating have actually allowed Vesta’s turbines to reduce the mass per MWh of energy produced:
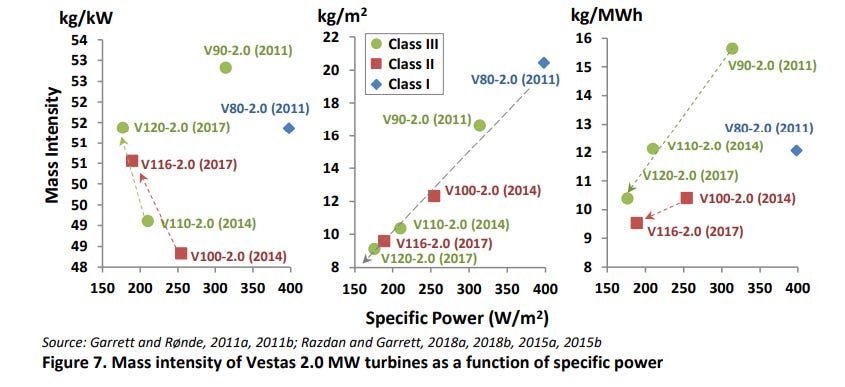
Wind turbines are thus a good illustration of the complexities and multifaceted nature of economies of scale. There are many simultaneous scale effects at work - learning curve effects, geometric scaling, statistical scaling, fixed cost effects - which often pull in different directions. It's not necessarily obvious, even to experts, which effects will dominate.
It's interesting to contrast the history of wind and nuclear power. Wind power scaled well. Larger wind turbines were cheaper per unit of power, more reliable and more efficient. Nuclear power scaled poorly. Larger plants were much more expensive to build as they grew larger and made it harder to control the damage of a catastrophic failure. We seem to have roughly topped out on wind turbine size, at least for land based turbines, with limits on construction and transportation of components. Meanwhile, we're seeing a next generation of smaller, modular nuclear plants that might make it easier to control costs and deal with failures.
Engineering is fascinating.
They don't fit the grid and they still kill off large birds, (unlike cats)